Rhizobium species: role in plant nutrition, crop quality, soil biology and climate change mitigation potential.
- Stanislav M.
- 2 days ago
- 33 min read
Updated: 6 minutes ago
Rhizobium spp. are saprotrophic soil bacteria best known for their symbiotic relationship with leguminous plants, where they fix atmospheric nitrogen into bioavailable form for plants nutritional needs.
In an era focused on sustainable agriculture and climate resilience, these microbes play a critical role by naturally fertilizing crops, improving soil health, and reducing the need for synthetic nitrogen fertilizers.
This report provides a detailed overview of how Rhizobium symbiosis works and its benefits, practical applications , considerations for field implementation, and how it aligns with current EU sustainability goals.
Symbiotic Nitrogen Fixation mechanism of Rhizobium
Rhizobium-Legume Symbiosis: Rhizobia (a collective term for Rhizobium and related genera like Bradyrhizobium, Sinorhizobium, Mesorhizobium, etc.) infect the roots of legumes to form specialized organs called nodules. The partnership is highly specific; particular Rhizobium strains nodulate specific legume hosts (for example, Rhizobium leguminosarum bv. viciae with peas, vetch, faba bean; Bradyrhizobium japonicum with soybean). In this symbiosis, both partners benefit: the plant supplies the bacteria with carbohydrates as energy, and the bacteria provide the plant with ammonia nitrogen converted from atmospheric N₂ . This natural exchange allows many legumes to thrive with minimal nitrogen fertilizer, and even enrich the soil for subsequent crops by leaving nitrogen-rich residues.
Nodule Formation Process: The establishment of rhizobial symbiosis is an intricate plant-microbe interaction. It begins with a molecular dialog in the rhizosphere. Legume roots under nitrogen starvation release signaling molecules (flavonoids) into the soil that attract compatible rhizobia.
In response, rhizobia synthesize Nod factors (lipochitooligosaccharides) that the plant recognizes, triggering the root hair to curl around the bacteria . The bacteria then initiate an infection thread – a tubular structure that penetrates root hair cells and guides the bacteria inward . Concurrently, cell divisions in the root cortex form a nodule primordium. The infection thread delivers rhizobia into these cortical cells, where they are released enclosed in a plant-derived membrane. This results in a root nodule, a new organ where the bacteria reside intracellularly. As nodules develop, the bacteria differentiate into specialized forms called bacteroids within plant cells.
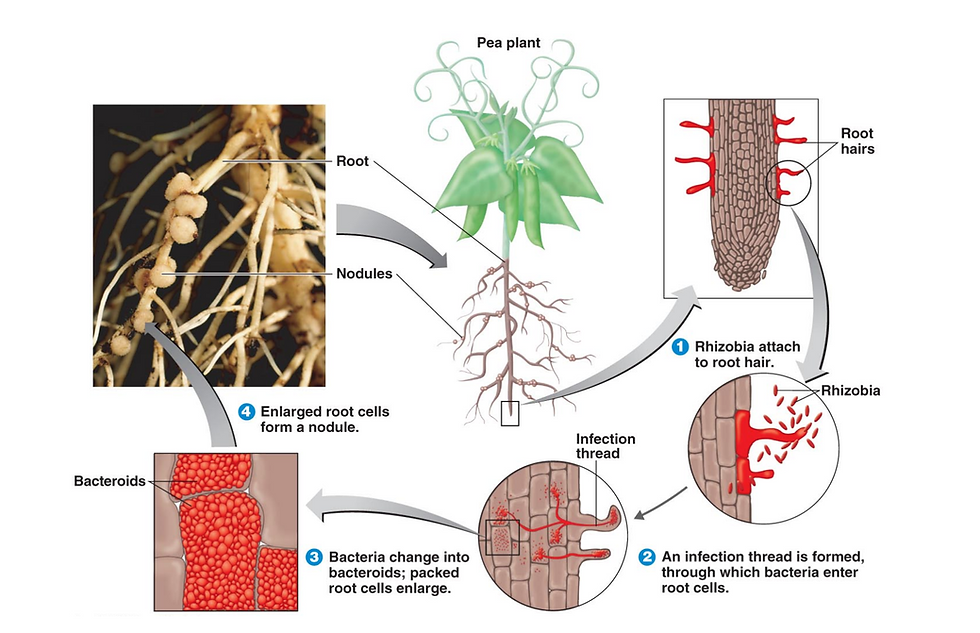
The plant tightly controls oxygen in the nodule (through leghemoglobin, which gives active nodules a pink/red color) to create a microaerobic environment required for nitrogen fixation.
The Nitrogenase Enzyme Complex: Inside the nodules, rhizobia express the nitrogenase enzyme complex – a two-component enzyme (dinitrogenase reductase and dinitrogenase) encoded by bacterial nif genes. Nitrogenase is the key catalyst that reduces atmospheric nitrogen (N₂) to ammonia (NH₃) using a large input of energy and reducing power. The overall reaction requires about 16 ATP and electrons per molecule of N₂, yielding ammonia and hydrogen as byproducts.
This is an energy-expensive process, which is why the symbiosis is so critical: the plant host feeds the bacteroids with energy-rich compounds (like malate) to drive nitrogen fixation.
Ammonia produced by nitrogenase is quickly assimilated by the plant into amino acids (e.g. glutamine), which become the building blocks of proteins and other vital molecules.
The end result is that legumes gain a self-sufficient nitrogen supply through their bacterial partners. This symbiotic nitrogen fixation can meet a large portion of the plant’s nitrogen needs – often 100–300 kg N/ha per year in crop systems – dramatically reducing or even eliminating the need for nitrogen fertilizer on that crop. For instance, faba bean in field trials obtained over 80% of its nitrogen from fixation and can leave significant residual nitrogen in the soil for the next crop .
Beyond Legumes – Other Associations: While Rhizobium mainly nodulates legumes, a few other plants can form similar symbioses. Notably, the tropical tree Parasponia (a non-legume) can be nodulated by rhizobia in a way akin to legumes. Moreover, some rhizobia can live as free-living or associative bacteria in the rhizosphere of non-legumes (grasses and other crops) and promote their growth via mechanisms like hormone production or minor nitrogen contributions. However, non-legume associations do not form true nitrogen-fixing nodules (with the special exception of Parasponia), so the primary agricultural application of Rhizobium remains with leguminous crops. That said, the Rhizobium-legume symbiosis is often leveraged in crop rotations and intercropping to benefit non-legume crops indirectly by improving soil nitrogen and microbiology.
Benefits of Rhizobium Symbiosis in Agriculture
1.Reducing Synthetic Nitrogen Fertilizer Inputs
One of the most impactful benefits of Rhizobium symbiosis is the reduction in requirement for inorganic nitrogen fertilizers. Legumes with effective Rhizobium nodulation can meet a large part of their N demand internally, which means farmers can apply little or no external N fertilizer to those crops. This directly saves on input costs and reduces environmental risks associated with fertilizers. Every kilogram of nitrogen fixed biologically is a kilogram that does not need to be produced via the energy-intensive Haber-Bosch process or applied as ammonia, urea, or nitrate in the field. By using efficient Rhizobium strains and compatible legume varieties, growers can significantly curtail the use of nitrogenous fertilizers ). In addition to on-farm savings, this has upstream environmental advantages: the production and distribution of synthetic fertilizers is a major source of greenhouse gas emissions and energy use, accounting for roughly 1–2% of global GHG emissions . Thus, replacing industrial fertilizer with biological nitrogen fixation (BNF) cuts down those emissions and the fossil fuel dependency of agriculture.
The benefits also extend to subsequent crops in rotation. Legumes often leave behind residual nitrogen in the soil in the form of crop residues, root fragments, and decomposed nodules. For example, a faba bean crop can not only supply most of its own N through BNF but also “leave economically valuable residual N for subsequent crops”
This residual nitrogen can reduce the fertilizer needs of the following cereal or other crop, a classic example of how including legumes in rotations improves nutrient cycling. In farming systems, this is an important strategy: farmers sowing legumes (like clover, peas, or beans) can improve soil N levels such that the next crop (wheat, corn, etc.) requires significantly less added fertilizer. Over time, this can build up soil fertility while decreasing synthetic fertilizer inputs year on year.
Beyond nutrients, there is a considerable climate change mitigation angle to reducing fertilizer use. Producing synthetic N fertilizer is energy-intensive (natural gas is the usual feedstock), and when applied to fields, excess fertilizer often leads to emissions of nitrous oxide (N₂O), a potent greenhouse gas. By relying on Rhizobium for N, farmers can both save energy and cut N₂O emissions. One review noted that encouraging Rhizobium-legume symbiosis in place of heavy fertilizer use offers clear benefits in “reducing greenhouse gas emissions and saving energy”.
Moreover, certain Rhizobium strains themselves can help mitigate greenhouse gases: some rhizobia possess a denitrification pathway including nitrous oxide reductase, meaning they can capture and convert N₂O (produced in soils) into harmless N₂ gas. In an in-situ field study, inoculation of soybean with a Bradyrhizobium strain carrying this N₂O-reductase enzyme cut soil N₂O emissions by about 70% compared to a standard strain. This demonstrates a direct emissions reduction potential by using the right inoculants. In summary, integrating BNF via Rhizobium can substantially reduce the need for synthetic fertilizers and the associated environmental footprint.
2.Enhancing Crop Yield and Quality
Effective Rhizobium inoculation has a well-documented positive impact on the yield and quality of legume crops. When a legume crop’s nitrogen demand is met through robust BNF, it typically produces higher biomass, more pods or seeds, and often higher protein content in the harvested product (thanks to ample nitrogen for protein synthesis). Many field trials and farm experiences attest to yield improvements from inoculation, especially on soils with no native rhizobial population or with suboptimal strains. For instance, a meta-analysis of legume inoculation trials found yield increases on the order of +20% to +60% in various contexts when effective rhizobia were provided.
In one case, cowpea yields across multiple studies increased about 1.5-fold (i.e., 50% higher) with the introduction of an effective Rhizobium strain ). Even in more moderate cases, single-digit percentage yield bumps can be economically significant given the low cost of inoculant. Farmers growing soybean in new areas of Europe, for example, have seen inoculation as essential – without it, nodules may not form and yields would be dismal, but with the correct Bradyrhizobium inoculant, soybean yields can reach their optimal potential (often a +30-50% yield improvement in previously uninoculated soils, according to agronomic reports).
It’s not just quantity – quality of produce also improves. Nitrogen is a key element in proteins, so nitrogen-fixing symbiosis often leads to higher protein levels in legume grains or forage. One study on peas (Pisum sativum) observed that Rhizobium inoculation enhanced seed yield (via better seed filling) and also increased seed protein content and overall quality.
The authors noted “the key role of Rhizobium as an effective nitrogen source for legumes’ seed quality and quantity improvement” in line with sustainable agriculture goals.
In essence, well-nodulated legume crops produce more nutritious seeds which is important for food/feed value. There are also cases where Rhizobium inoculation helps the crop mature more uniformly (due to improved nutrition), resulting in more consistent seed size and quality.
It should be noted that the yield benefit of inoculation can depend on the context. In soils that have grown a particular legume for many years, there may already be abundant native rhizobia that nodulate the crop effectively. In those cases, adding more rhizobia (via inoculant) might not show a large yield boost, as the baseline BNF is already occurring. For example, long-term cultivation of clover or beans in an area often means the soil carries a persistent rhizobial population (often 10^5–10^6 viable rhizobia per gram of soil in some European fields , capable of nodulating the next crop. However, when introducing legumes into new fields or regions (e.g., expanding soybeans into northern Europe, or chickpea into areas it wasn’t grown before), inoculation with the appropriate Rhizobium strain is critical to ensure nodulation and avoid yield penalties. Even in soils with some native rhizobia, using elite inoculant strains can sometimes outperform the indigenous bacteria, leading to better nitrogen fixation and higher yields.
Thus, to maximize legume yield and quality, it’s important to assess the need for inoculation and choose high-quality strains known to be efficient nitrogen fixers.
3.Improving Soil Biology and Microbiome Health
Rhizobium symbiosis contributes to soil health in multiple ways. First, by fixing nitrogen and enhancing plant growth, legumes under Rhizobium inoculation increase the return of organic matter to soil (through leaf drop, root turnover, and crop residues). This organic matter feeds soil organisms and improves soil structure. Over time, legume rotations are known to build up soil organic carbon and aggregate stability, which benefits the overall soil microbiome. The presence of legumes can increase microbial biomass in soil and promote a more diverse microbial community compared to continuous cereal cropping. This is partly because legumes exude different compounds (including those flavonoids and other signals) that stimulate microbial activity in the rhizosphere.
Rhizobium itself is a beneficial microbe added to the soil (when inoculated) – it becomes part of the soil microbial community. Unlike chemical inputs, which might disrupt microbial balances, using microbial inoculants works with the soil biology. Soil biodiversity tends to improve when farmers integrate legumes; an EU research agenda highlights that legume crops provide ecosystem services “including those related to soil biodiversity and fertility”.
Healthy populations of rhizobia and other symbionts can crowd out or suppress certain soil pathogens by competition or by inducing plant resistance mechanisms. In fact, Rhizobium has been shown to have plant-protective effects beyond just supplying nitrogen. Studies indicate that legumes nodulated by Rhizobium experience a form of “priming” of their immune system, leading to enhanced resistance against some diseases and stresses.
For example, in peas infected by a fungal pathogen (Didymella pinodes causing ascochyta blight), Rhizobium-inoculated plants had significantly lower disease severity and higher seed yields compared to uninoculated plants.
Rhizobium symbiosis had triggered changes in the plant (detected via proteomics and metabolomics) that bolstered the pea plant’s defenses, resulting in less pathogen damage. This kind of induced systemic resistance means Rhizobium inoculation can indirectly reduce the need for certain pesticides and improve plant health.
In terms of the soil food web, growing legumes benefits subsequent crops by nurturing beneficial microbes. Many farmers observe that a good clover or bean crop leaves the soil “in good heart” – looser, richer in earthworms and microbes – for the next planting. Part of this is due to nitrogen enrichment, but also the root system differences (legumes often have deep taproots or abundant root networks that improve porosity). Additionally, decaying nodules release not just nitrogen but also trace elements (like molybdenum and cobalt that were concentrated for enzyme use) and polysaccharides that can act as soil glues. The net effect is a more biologically active and fertile soil. In essence, Rhizobium-legume symbiosis acts as a natural biofertilizer, feeding not just the crop but the soil ecosystem. It aligns with regenerative agriculture principles that seek to enhance soil life. When combined with reduced chemical fertilizer inputs, this can avoid the negative impacts that excess soluble nutrients sometimes have on soil microbial balance (e.g. reducing mycorrhizal fungi when too much N is added). Thus, Rhizobium helps steer the system toward a self-sustaining, biologically rich fertility cycle.
Climate Change Mitigation Contributions
The use of Rhizobium in agriculture contributes to climate change mitigation in several ways. A primary contribution is through the reduction of greenhouse gas emissions associated with synthetic nitrogen fertilizers. As discussed, manufacturing fertilizer is carbon-intensive (emitting CO₂), and applying it can cause emissions of N₂O. By fixing nitrogen in planta, Rhizobium symbiosis avoids a portion of these emissions. A review paper concluded that deploying effective Rhizobium strains to replace some fertilizer can reduce the “energy inputs and greenhouse gas emissions” from agriculture.
This is directly supportive of global climate goals. For example, if a farming region replaces 50% of its synthetic N use with legume BNF, the emissions savings are substantial – both from factories and from fields.
Another angle is that well-nodulated legume crops often have a lower carbon footprint per unit of yield. This is important for life-cycle assessments of crop production. A ton of soybean produced with all its N coming from BNF has a much smaller CO₂-equivalent emission than a ton of non-legume grain produced with heavy synthetic N. Some rhizobial inoculants are being promoted as carbon farming tools, where farmers can potentially earn carbon credits for reducing fertilizer-related emissions by planting nitrogen-fixing crops.
Additionally, integrating legumes can enhance carbon sequestration in soils. Legume residues (especially from perennial forage legumes like alfalfa or clover) contribute to soil organic carbon. Healthy, biologically active soils (as fostered by Rhizobium and legumes) can lock away more carbon over time.
While the primary climate benefit of Rhizobium comes from N-related emission reductions, this carbon sequestration co-benefit is also valuable for climate resilience.
It’s also worth noting the synergy with climate adaptation: legumes and their rhizobia can make farming systems more resilient to shocks. For instance, during fertilizer shortages or price spikes (as seen recently), farmers who can rely on nitrogen-fixing crops are less vulnerable. This “nutrient self-sufficiency” contributes to food security under climate and market volatility.
Finally, as mentioned, certain Rhizobium strains can mitigate nitrous oxide emissions in situ. This is an active area of research: scientists are exploring inoculants that not only fix N₂ but also consume N₂O produced by soil microbes or fertilizer. The earlier example of a strain achieving 70% less N₂O in soybean fields (New Insights into the Use of Rhizobia to Mitigate Soil N 2 O Emissions) is promising for greenhouse gas mitigation. Such microbial solutions are attractive as they leverage natural processes to tackle emissions that are otherwise hard to manage on farms.
Plant-Microbe Interaction Dynamics and Stress Resilience
The legume-Rhizobium symbiosis is sensitive to environmental conditions. Achieving optimal nodulation and nitrogen fixation requires attention to certain soil and agronomic factors:
Soil Nitrogen Levels: Interestingly, too much available nitrogen in soil (from fertilizer or manure) can suppress the symbiosis. Legumes will preferentially take up mineral nitrogen first and may down-regulate nodule formation if plenty of N is readily available. High levels of nitrate in soil can delay or reduce nodulation and can even inhibit the activity of nitrogenase in existing nodules. Essentially, the plant “decides” it doesn’t need to pay the cost of feeding rhizobia if it can get free N from soil. Farmers must be aware that adding fertilizer N to legume crops can be counterproductive – it’s usually recommended to either avoid N fertilizer or apply only a small “starter” dose at planting if necessary, to encourage the plant to fully engage with Rhizobium. Over-fertilization not only wastes inputs but could result in a well-grown legume that ironically isn’t fixing much N because it didn’t bother to nodulate. This balance is important in management.
Soil pH: Most Rhizobium species prefer neutral to slightly alkaline soils for best performance. Acidic soils (low pH) are a common obstacle to good nodulation. In low pH conditions, rhizobia survival and movement can be poor, and the chemical signaling may fail. Some rhizobia are particularly sensitive to acidity, and the legume root hairs also respond differently. For example, Rhizobium leguminosarum (peas, beans) does not thrive if soil pH drops too low (below ~5.5). In acidic soils, farmers might need to apply lime to raise pH or use specially selected acid-tolerant Rhizobium strains. There are documented successes in this area: In field trials with faba bean on acidic soils, an acid-tolerant inoculant strain (e.g., Rhizobium sp. strain SRDI-969) boosted nodulation by 65% and increased yields by ~24% compared to the standard strain. This shows that matching the Rhizobium strain to soil conditions is possible (via strain selection in inoculants) to overcome pH challenges.
Temperature: Soil temperature affects nodule formation. Early in the season, cold soils can slow down Rhizobium activity and nodule development. Some Bradyrhizobium (for soybean) are less effective under cold conditions, which is why soybean traditionally was grown in warmer climates; breeding of both soybean and its rhizobia is ongoing to extend nitrogen fixation to cooler climates. Extremely high soil temperatures (above 35°C) can also harm the symbiosis, as the enzymes and root interactions get disrupted. However, many rhizobia can adapt if the high temperatures are not constant.
Moisture and Aeration: Adequate soil moisture is needed for rhizobia mobility and survival (they move in soil water to reach roots). Drought can limit nodulation or cause nodule abortion as the plant under stress might not support the bacteria. Waterlogged conditions, on the other hand, create anaerobic soil which can kill rhizobia or prevent infection. Good drainage and irrigation practices help maintain the moderate moisture that benefits nodulation. Interestingly, nodules themselves require a low-oxygen environment internally (for nitrogenase) but the soil around roots should be well-aerated to allow normal root respiration and bacterial activity. Compacted or waterlogged soils can thus indirectly suppress BNF.
In summary, to maximize the Rhizobium-legume interaction, farmers should ensure a conducive soil environment: not too much inorganic N, pH near neutral if possible, and proper moisture and aeration.
Stress Tolerance and Resilience
An exciting aspect of Rhizobium symbiosis is its contribution to plant stress tolerance. Research has shown that nodulated legumes often handle stresses (like drought or salinity) better than if they were not nodulated . Part of this is simply due to improved nutrition – a well-fed (nitrogen-sufficient) plant is generally healthier and more stress-resilient. But beyond that, Rhizobium can actively help the plant cope with stress through various mechanisms:
Drought: Some Rhizobium strains induce physiological changes in their host that improve drought tolerance. For example, inoculation of certain drought-tolerant rhizobia in crops has been linked to increased accumulation of osmoprotectants (like proline or trehalose) in the plant, better root architecture (deeper roots to find water), and improved stomatal behavior under water stress. Studies on common bean and other legumes found that plants with drought-adapted rhizobia continued nitrogen fixation longer into a dry period and recovered faster when re-watered. In some cases, rhizobia also produce exopolysaccharides that improve soil structure around roots, helping retain moisture.
Salinity: Soil salinity is notoriously harmful to both plants and soil microbes. Yet, certain rhizobia confer greater salt tolerance to their legume hosts. They may do this by producing enzymes and compounds that mitigate salt stress – for instance, rhizobial production of antioxidant molecules can reduce oxidative stress in plants under high salinity.
In a study on common beans, rhizobia helped maintain better ion balance (K⁺/Na⁺ ratios) in the plant tissues and kept photosynthetic rates higher under salt stress. Some strains are inherently salt-tolerant and can nodulate even when salt levels are high, thereby continuing to supply nitrogen when the plant might otherwise be starving in salty soils. Using such salt-tolerant inoculants is a strategy in coastal or arid-region agriculture.
Diseases and Pests: As mentioned earlier, rhizobial symbiosis can induce systemic resistance in the host plant. The presence of rhizobia triggers the plant’s immune system in a way that prepares it to fight off certain pathogens more effectively (this phenomenon is somewhat analogous to a vaccine effect for plants). Beyond the pea disease example (Frontiers | Rhizobium Impacts on Seed Productivity, Quality, and Protection of Pisum sativum upon Disease Stress Caused by Didymella pinodes: Phenotypic, Proteomic, and Metabolomic Traits), other studies have shown reduced root rot or wilt incidence in nodulated legumes compared to nitrogen-fertilized controls. Some rhizobia also produce antifungal compounds in the rhizosphere or compete with pathogens for space and resources on the root. While Rhizobium is not primarily used as a biocontrol agent, these ancillary benefits are welcome in integrated pest management. A healthier plant can also better resist insect pests, and higher protein content in tissues (from good N nutrition) sometimes deters certain insects.
General Resilience: Legumes in rotation can break pest and disease cycles for subsequent crops (a rotational benefit), and their improved soil structure can help mitigate effects of erosion or heatwaves on the field. Collectively, a farming system that incorporates Rhizobium and legumes tends to be more resilient to climate extremes – e.g., after a drought, a field with a history of legumes might recover soil function faster thanks to better organic matter and microbial networks.
It’s important to pair the right Rhizobium strain with the right legume to achieve these stress tolerance benefits. Scientists are increasingly isolating and testing stress-tolerant rhizobia – for instance, strains from arid regions for use in drought-prone farms, or strains from alkaline/saline soils for similar conditions. The symbiosis itself must endure stress to continue fixing nitrogen; thus, strains that can withstand stress (and keep fixing N) are valuable. The combination of a tolerant strain and a tolerant crop variety can substantially improve yields under stress conditions compared to non-inoculated or sensitive pairings. In practice, this means that inoculant producers and researchers are tailoring biofertilizers for climate resilience, which is a promising tool as we face more erratic weather and challenging growing conditions.
Synergy with Other Soil Microbes (Mycorrhizae and More)
Rhizobium does not work in isolation in the soil; it often interacts with other beneficial soil microbes to the plant’s advantage. One of the most important partnerships is with arbuscular mycorrhizal fungi (AMF). Legumes can form a tripartite symbiosis: plant–rhizobia–mycorrhizae. The AM fungi colonize the plant’s roots and assist with phosphorus and micronutrient uptake, while rhizobia handle nitrogen; together they complement each other’s functions. Numerous studies have shown that co-inoculating legumes with Rhizobium and mycorrhizal fungi yields better outcomes than either alone. In fact, a meta-analysis found “strong synergistic effects of AMF and rhizobia inoculation on plant biomass production” – plants grew significantly larger when both symbionts were present, beyond what would be expected from nutrient improvement alone . The synergy arises because nitrogen fixation is an energy-intensive process that also requires plenty of phosphorus (for ATP and nucleic acids).
Mycorrhizae greatly increase phosphorus uptake for the plant, which in turn supports more active nitrogen fixation in the nodules. Meanwhile, the additional nitrogen from rhizobia can help the plant grow more roots for the fungi and produce more carbon to feed both symbionts. It’s a win-win-win situation among the three parties.
Another interesting finding is that mycorrhizal fungal hyphae can actually help distribute rhizobia in the soil and bring them closer to plant roots. AMF hyphae exploring the soil can transport or attract rhizobia, effectively increasing the encounter rate between rhizobia and legume roots.
This can lead to earlier or more effective nodulation. In one experiment, the presence of the mycorrhizal fungus Rhizophagus irregularis helped Sinorhizobium meliloti bacteria reach the roots of Medicago plants more efficiently, resulting in increased nodule formation (essentially acting like highways for bacteria). This fascinating cross-talk suggests that designing consortia of microbes could optimize overall symbiosis.
Beyond mycorrhizae, Rhizobium can also synergize with other plant growth-promoting rhizobacteria (PGPR). Co-inoculation of legumes with rhizobia and certain beneficial bacteria (such as Pseudomonas, Bacillus, or Azospirillum) often shows additive or synergistic effects. For example, a common bean trial with both Rhizobium and a phosphate-solubilizing bacteria saw higher nodulation and yield than with Rhizobium alone, because the co-inoculant increased phosphorus availability to the plant (similar in effect to mycorrhiza). In another instance, combining Rhizobium with a biocontrol fungus like Trichoderma improved plant growth and health – Trichoderma helped control root pathogens while Rhizobium provided nitrogen, together boosting legume biomass. Researchers have noted that such “biocompatible inoculant mixtures” can have “strong synergistic relationships” that multiply plant growth benefits. However, they also caution that combining too many microorganisms at once can lead to competition that diminishes their effectiveness.
For instance, a triple inoculation (Rhizobium + AMF + a bacterium) might perform worse than a double inoculation if the microbes compete for root space or exudates. Therefore, selecting complementary organisms and verifying their compatibility is important for successful multi-microbe products.
In practical farming, these synergies mean that using Rhizobium inoculant doesn’t preclude other biological inputs – in fact, pairing Rhizobium with mycorrhizal inoculants or organic soil amendments can produce a more robust crop response. Many modern “biofertilizer” formulations contain Rhizobium mixed with other beneficial microbes to target multiple plant needs. For example, some pea and lentil inoculants in the market now include both nitrogen-fixing bacteria and mycorrhizal spores. The goal is to offer farmers a convenient package that addresses N, P, and disease protection biologically. When applied properly, these combinations help create a healthy soil microbiome where microbes support each other’s functions and collectively enhance plant growth. This approach aligns with the concept of treating the soil as a living ecosystem – fostering the right consortium of microbes can amplify the natural processes that sustain plant productivity and resilience.
Field Successes in Different Agroecosystems
Rhizobium inoculation is a well-established practice in many agricultural systems around the world. One classic example is soybean production. In regions like North and South America, soybeans (a legume) are grown at massive scales, and farmers routinely inoculate soybean seeds with Bradyrhizobium strains before planting. This practice has enabled high yields (2–4 tons/ha) on soils that would not naturally have the appropriate rhizobia. Brazil’s soybean boom, for instance, was underpinned by successful inoculation programs – tropical Brazilian soils initially lacked soybean-specific rhizobia, but scientists introduced effective strains (like Bradyrhizobium elkanii) and developed inoculant industries, allowing soy cultivation to expand without proportional fertilizer increases. This has saved Brazil billions of dollars in fertilizer costs and avoided untold environmental damage, making it a hallmark success story in applied BNF. Similarly, in North America, modern soybean varieties are paired with improved inoculant strains; even where soy was grown before, periodically inoculating with elite strains can refresh the soil population and ensure top performance.
In Africa and Asia, various bean, pea, and groundnut (peanut) inoculation projects have shown remarkable yield gains, especially on smallholder farms with poor soil fertility. As mentioned earlier, cowpea and groundnut in West Africa responded with over 50% yield increases in some cases when inoculants were provided . Such results are transformative for subsistence farmers, turning a near-failing crop into a productive one. In India, Rhizobium inoculants for chickpea and pigeonpea are widespread, and government programs distribute packets of inoculum to farmers as a low-cost aid for improving pulse production. These are examples outside Europe, but they underscore the universal relevance of Rhizobium.
European Context: In Europe, the use of Rhizobium is gaining renewed attention due to sustainability goals and an increasing interest in protein crops. Historically, Europe has grown legumes like peas, faba beans, alfalfa, clover, and lupins, and many soils do contain native rhizobia for these traditional legumes (owing to decades or centuries of cultivation and naturalized populations). For example, studies in the UK and northern Europe found that even fields with no recent history of legumes still had Rhizobium leguminosarum bacteria present at high enough levels to nodulate a faba bean crop. This is likely due to wild or volunteer legumes and the hardiness of rhizobia that persist in soil seed banks. As a result, some farmers in long-established agricultural areas might not always inoculate peas or beans, especially if previous tests showed adequate nodulation from native soil bacteria. However, with the introduction of new legume species (like soybean) or in regions where certain legumes were not common, inoculation is essential.
Soybean is a case in point for Europe: Soy cultivation is expanding in central and southern Europe (e.g. Italy, France, Romania, Ukraine) as part of efforts to produce more protein crops locally. European soils typically lack the specific Bradyrhizobium japonicum strains needed by soybeans, so inoculating soybean seed is a must for any farmer attempting the crop. Over the past decade, European agronomy trials with soybean inoculants have shown very positive results, enabling soybeans to yield competitively (e.g., 2.5–3.5 t/ha) under European conditions when properly inoculated. In Italy, for instance, where soybean is now well-established, the inoculant market has grown rapidly, and multiple strains (including some European-developed Bradyrhizobia) are available to farmers. The success of soybean in non-traditional areas is often cited as a modern testament to the power of microbial inoculation in unlocking a crop’s potential.
Another European example is in the improvement of forage legumes. Countries like Ireland, the Netherlands, and Denmark rely on clover in pasture to provide natural nitrogen for grass (mixed grass-clover swards are common to reduce fertilizer on dairy farms). While clovers naturally find rhizobia in soil, research projects have looked at introducing more effective strains to further boost clover N₂ fixation. Some trials indicate that new inoculant strains can increase clover biomass and the total N fixed per hectare, though results vary depending on how competitive the native strains are.
Large-scale demonstrations have also been conducted for peas and faba beans. In Eastern Europe (e.g., Poland, Ukraine) and parts of Russia, inoculating peas with Rhizobium leguminosarum has been shown to increase yields and grain protein content, particularly on land that had been out of pulse production for a long time (e.g., former wheat monocultures). European farmers are also exploring new legume crops like lupins, cowpeas (in the south), and chickpeas – all of which require matching inoculants. For lupins, specific Bradyrhizobium strains are needed; for chickpea, Mesorhizobium ciceri is used. As these crops are promoted for diversification, ensuring the right rhizobial partners is part of the package.
An interesting case study comes from organic farming. Organic systems, which avoid synthetic N fertilizers, lean heavily on legumes for fertility. Many organic rotations include a legume cover crop or fertility-building phase (e.g., a year of alfalfa or clover). The performance of these legumes can determine the success of subsequent cash crops. In Europe, some organic farms have started inoculating even cover-crop legumes to guarantee strong nodulation, especially if soil conditions are tough or if they’re using a legume species new to the farm. For example, an organic farmer in Germany might inoculate vetch or field peas when using them as a winter cover, to maximize N fixation over the winter and thus provide more nitrogen to the spring crop. This is a low-cost insurance to make sure the cover crop fixes the nitrogen it’s supposed to.
In terms of quantifiable benefits, a well-nodulated legume can fix substantial nitrogen: values of 100–200 kg N/ha for pea or bean crops, and up to 300+ kg N/ha for vigorous alfalfa or clover stands over a year, have been reported in Europe. Not all of this becomes immediately available to the next crop (much is tied in the legume biomass), but even the portion that is mineralized can cut fertilizer needs significantly. Furthermore, those legumes contribute to yield stability; for instance, in dry years, a pea crop with Rhizobium might outyield a fertilized cereal crop because the pea can continue to get N via fixation when soils are too dry for fertilizer uptake.
In summary, real-world usage of Rhizobium ranges from small farmers coating seeds with inoculum on-site, to large operations where seed comes pre-inoculated from the supplier. There have been success cases across diverse agroecosystems: from Canadian prairies (lentils and peas) to African savannas (cowpea, groundnut) to European farmlands (faba, soy). Each case underscores how a tiny bacterium can have outsized impacts on productivity and sustainability. While results can vary with context, the overarching narrative is that leveraging biological nitrogen fixation is both agronomically and economically beneficial when done correctly.
Challenges and Considerations for Implementation
Implementing Rhizobium-based solutions in the field comes with some practical challenges and points to consider. To reap the full benefits discussed, farmers and practitioners must navigate these considerations:
1. Soil and Environmental Constraints: As noted, factors like pH, nutrient levels, and climate can influence Rhizobium performance. If a soil is very acidic or deficient in certain nutrients (e.g., phosphorus, molybdenum, or calcium), simply adding rhizobia might not result in great nodulation or fixation. It may be necessary to amend the soil (apply lime to raise pH, or ensure adequate P and micronutrients) in conjunction with inoculation. For example, molybdenum is a cofactor for the nitrogenase enzyme; in some tropical soils Mo is low, and farmers apply a seed coating of molybdenum along with Rhizobium inoculant to ensure the bacteria can function properly. Similarly, if soil is extremely deficient in organic matter, building it up over time will help the introduced rhizobia survive and thrive. Drought or heat during the growing season is another challenge – if nodules experience severe stress, the fixation process can slow or stop. In such cases, irrigation (if available) or mulching to conserve moisture can indirectly support the symbiosis. Essentially, the better the overall soil health and conditions, the better the Rhizobium symbiosis will work. As part of extension advice, agronomists often include nodulation checks (examining roots for nodules) in their crop scouting; if nodulation is poor, they diagnose whether soil conditions might be the cause (e.g., “Was there too much residual N?” or “Is the soil waterlogged?”) and recommend corrections either in-season or for next time.
2. Crop-Rhizobium Specificity: It’s critical to use the right Rhizobium strain for the target crop. There is not a one-size-fits-all inoculant for all legumes. Legumes are grouped into cross-inoculation groups – for instance, peas, lentils, vetch, and faba bean share similar rhizobia (R. leguminosarum biovar viciae), whereas soybeans require Bradyrhizobium, and chickpeas need Mesorhizobium. Using the wrong type will result in no nodulation (or ineffective nodules). Farmers must ensure they purchase the correct inoculant specified for their crop (in practice, inoculant products are labeled clearly by crop). In mixed legume stands (say a cover crop mix containing clover, vetch, and pea), multiple rhizobia might be needed if they are from different groups, but fortunately many common legumes do overlap in their rhizobia requirements. Crop compatibility also extends to varieties: most modern legume cultivars nodulate readily, but occasionally a new variety might have slightly different preferences or less nodulation if not matched with the optimal strain. Plant breeders typically ensure that any new legume variety is tested with available inoculants. There have been rare cases of incompatibility (e.g., a peanut variety that nodulates poorly with a standard strain), which underscores the need for ongoing evaluation and perhaps inoculant updates.
Another consideration is crop rotation and previous legumes: If the same legume is grown repeatedly, specific rhizobia will accumulate in soil. But if a legume hasn’t been grown for many years, the specific rhizobia might be absent. For example, if a farmer last grew soybeans 15 years ago, it’s prudent to inoculate again now because the population could have dwindled or lost effectiveness. Conversely, if clover has been in a pasture for a long time, soil will be rich in clover rhizobia, and adding more might not change much. Understanding these dynamics can guide whether inoculation is necessary each season or if the soil has a sufficient natural reservoir. As a rule of thumb, when in doubt, inoculate – the cost is low relative to the potential benefit, and it poses no harm even if rhizobia were already present.
3. Inoculant Quality and Handling: The efficacy of Rhizobium inoculation heavily depends on the quality of the inoculant product and proper application. Rhizobium inoculants are living products – typically cultures of the bacteria formulated on a carrier (such as peat, clay, or liquid). Key considerations include:
Strain efficacy: Not all strains are equal. Farmers should use inoculants from reputable suppliers that contain proven, effective strains for their crop. In the EU and many countries, strains are tested and approved for commercial use. For example, Rhizobium leguminosarum might be included in pea inoculant because it’s known to fix well. Using high-performance strains can be the difference between fixing 50 kg N/ha versus 150 kg N/ha in a season.
Viability: The product must contain a high number of live rhizobia at the time of application. Many inoculants guarantee a minimum count (often around 10⁹ cells per gram). Achieving a good infection requires delivering enough rhizobia to each seed. Studies suggest aiming for at least around 10⁵–10⁶ viable rhizobia per seed at planting for reliable nodulation. Handling is crucial: inoculant should be stored in cool conditions (refrigerated if possible) and used before its expiration date. Heat or direct sunlight can kill the bacteria. It’s also important to avoid drying out – once applied to seed, the seed should be planted within a reasonable time, and not left in the hot sun for hours. Proper storage and use of fresh inoculants maximize their efficacy.
Application method: Inoculants can be applied as a seed coat (most common), as a planter box treatment, or in-furrow to the soil. Seed coating (either done on-farm or by seed companies pre-inoculating seed) ensures the rhizobia are right where the emerging root will be. When coating seeds, using adhesives or sticking agents can help the powder adhere. Farmers must also consider if the seeds are treated with pesticides: some fungicide or insecticide seed treatments can harm rhizobia on the seed. There are now inoculant formulations compatible with treated seed (using protective polymers or tolerant strains), but it’s always advisable to check compatibility. Often, the recommendation is to inoculate as close to planting as possible, especially if fungicide-treated seed is used, or to use double the inoculation rate to offset any losses. In-furrow granular or liquid inoculants are an alternative; these deliver rhizobia into the seed furrow in the soil. They can be beneficial in dry conditions (providing a moist carrier environment in the furrow) or when farmers prefer not to handle treated seed.
Competition with native strains: In soils that have native rhizobia populations (even if suboptimal fixers), there is a competition for nodule occupancy. Sometimes an introduced inoculant strain might be very efficient at fixing N, but it could be a poor competitor and gets outcompeted by less efficient native strains that colonize the nodules instead. This is a subtle but important challenge – it means that just because you apply a great strain doesn’t always guarantee it will dominate in nodules. Breeding “competitive and effective” strains is a goal for inoculant developers. One strategy to deal with this is using high inoculation rates (flood the root zone with so many of the good rhizobia that they win by sheer numbers). Another approach is strain improvement to give them an edge in survival or root colonization. Farmers may not directly see this competition, but they might observe year-to-year differences in performance if, say, an inoculant worked great the first year (when soil was naive) but then in later years native strains took over. Monitoring nodule effectiveness (nodules should be pink/red inside, indicating active leghemoglobin and N-fixation) can give a clue – if nodules are white or green inside, they might be ineffective (perhaps due to a poor strain). In such cases, switching to a different inoculant strain or addressing soil issues might be needed.
Regulatory quality control: In the EU and many regions, microbial inoculants are subject to quality regulations. The EU has updated its fertilizing products regulation (Regulation (EU) 2019/1009) to include biostimulants and biofertilizers which cover Rhizobium inoculants. This means products should meet certain standards for microbial content, absence of contaminants, and efficacy claims. Farmers should use registered products to ensure they are getting what is promised. Poor-quality or improperly produced inoculants (with low counts or wrong strains) can lead to disappointing results and undermine confidence in the technology.
4. Integration into Farming Systems: For successful implementation, Rhizobium inoculation should be integrated with the crop management plan. Timing of planting, seedbed preparation, and subsequent field operations all play a role. For example, if a field is very dry at planting, even with inoculation the nodulation might be delayed until rains come (because bacteria need moisture to move to roots). Irrigating after planting could help establishment of the symbiosis in such cases. If a farmer plans to apply herbicide, there is generally no issue (most herbicides don’t affect underground bacteria), but soil-applied herbicides or fertilizers (like starter phosphorus placed near seed) should be placed so as not to harm the inoculant – salt injury from fertilizers can kill bacteria just as it can damage seedlings.
From an operational perspective, many farmers find inoculation to be easy and routine, but it does require an extra step. For larger operations, purchasing pre-inoculated seed or using planter attachments for granular inoculant can streamline the process. For smallholders, mixing inoculant slurry with seeds in a bucket on planting day is common. Education and training ensure that this is done correctly (for instance, using non-chlorinated water if making a slurry – since chlorine can kill bacteria). Outreach programs often demonstrate the nodulation results to farmers by digging up sample plants mid-season. Seeing the pink nodules on roots gives farmers confidence that the practice is working and encourages them to continue it.
In summary, while using Rhizobium inoculants is generally straightforward and low-risk, attention to detail can make the difference between a spectacular result and a mediocre one. Farmers should treat inoculants as living organisms – handling them carefully – and agronomists should tailor recommendations to local conditions (right strain, soil amendments if needed, correct application). When these considerations are addressed, the probability of achieving successful, nitrogen-rich legume crops is very high.
Policy and Sustainability Initiatives Supporting BNF in Europe
The push for Rhizobium use and biological nitrogen fixation in agriculture is not only a grassroots or scientific effort – it is increasingly backed by policy and sustainability initiatives, especially in the European Union. Several high-level strategies recognize the value of legumes and BNF in creating a more sustainable and climate-friendly food system:
European Green Deal and Farm to Fork Strategy: The European Green Deal, launched in 2019, is the EU’s roadmap for making the economy sustainable, and within it, the Farm to Fork Strategy specifically targets agriculture. The Farm to Fork Strategy sets ambitious goals for reducing chemical inputs: notably a 20% reduction in fertilizer use by 2030 (along with a 50% reduction in pesticide use).
Achieving a cut in fertilizers while maintaining productivity implies relying more on natural processes like biological N fixation. The strategy explicitly mentions the need to increase the availability of alternative protein sources, including plant proteins, and notes that attaining the fertilizer reduction target “will create a favourable environment for the development of EU-grown protein plants which naturally enrich the soil, reducing the need for synthetic fertilisers” (Valorisation of ecosystem services provided by legume crops | Horizon-europe.gouv.fr) .
In other words, the EU sees expanding legume cultivation as a key step toward those environmental targets. The Farm to Fork Strategy and the EU Biodiversity Strategy also highlight legumes for their role in diversifying cropping systems and delivering ecosystem services.
Common Agricultural Policy (CAP) Reforms: The CAP 2023–2027 has introduced new mechanisms to encourage sustainable farming, including eco-schemes which are voluntary practices farmers can adopt for additional payments. Many EU countries’ CAP Strategic Plans include eco-schemes related to planting legumes or cover crops due to their environmental benefits. For instance, there are eco-schemes for maintaining a percentage of land in nitrogen-fixing crops or using leguminous cover crops over winter. The new CAP explicitly supports “longer rotation cycles with environmentally beneficial crops such as leguminous crops” (Valorisation of ecosystem services provided by legume crops | Horizon-europe.gouv.fr). This is meant to incentivize farmers to integrate legumes into their arable rotations, thereby harnessing BNF. Other CAP instruments benefiting protein crops include coupled payments in some countries (direct subsidies per hectare for growing protein crops like peas, beans, soy) and rural development programs that fund demonstration projects or equipment (like specialized planters or inoculation equipment). The motive is both to reduce dependency on imported protein (soymeal) and to gain the environmental upsides of more legumes in European fields. The CAP’s conditionality also has standards (GAEC - Good Agricultural and Environmental Conditions) that encourage crop diversification; one GAEC standard (GAEC 7 crop rotation and GAEC 8 ecological focus areas) specifically mentions legumes and nitrogen-fixing crops as options to fulfill those requirements (Nature and Nitrogen - CAP battles over Conditionality) (Nature and Nitrogen - CAP battles over Conditionality). Essentially, the policy framework is aligning to make legumes + Rhizobium an attractive choice for farmers, rewarding them for providing public goods like soil fertility and climate mitigation.
European Legume Initiatives:
In recent years, there have been EU-funded research and innovation projects aimed at boosting legume cultivation and utilization. Projects like “LEGUME HUB”, “TRUE” (Transition Paths to Sustainable Legume-based systems in Europe), and others bring together researchers, industry, and farmers to share knowledge on best practices for legumes and their symbionts. These platforms disseminate findings on which inoculant strains perform best in which region, how to manage legumes in organic systems, and how to breed both better legumes and better rhizobia. The EU’s Horizon research programs have calls focusing on protein crops and their ecosystem services
(Valorisation of ecosystem services provided by legume crops | Horizon-europe.gouv.fr) .
For example, a Horizon 2020 project looked at breeding pea varieties that can fix more nitrogen or that nodulate more effectively under stress, while another studied the “valorisation of ecosystem services provided by legume crops” to quantify benefits like improved soil biodiversity (implicitly, the role of microbes like rhizobia). The expected outcomes of these initiatives include greater knowledge and capacity for farmers to successfully include legumes in cropping systems and thereby achieve positive ecological and economic impacts (Valorisation of ecosystem services provided by legume crops | Horizon-europe.gouv.fr).
National Policies and Green Deals: Some EU member states have their own targets or strategies that dovetail with the EU’s goals. For instance, the Netherlands has a Protein Strategy to increase domestic protein crop production. France had a “Protein Plan” aiming to boost legumes to reduce soy imports. These often emphasize inoculation and BNF as critical to making protein crops competitive and sustainable. Additionally, given the current geopolitics (e.g., the war in Ukraine causing fertilizer and feed shortages), there is heightened attention on biological nitrogen sources.
EU officials and MEPs have argued for sowing more nitrogen-fixing crops in response to fertilizer scarcity and high prices, noting that this would increase resilience and self-sufficiency. There’s recognition that legumes can help buffer Europe from external shocks in fertilizer supply, reinforcing the need for supportive policies.
Environmental Regulations: On the flip side, regulations like the Nitrates Directive (which limits excessive fertilizer application in vulnerable zones) indirectly encourage finding alternative nitrogen sources – again pointing to legumes. The push to reduce agricultural N runoff and water pollution (to meet Water Framework Directive goals) also makes a case for biological N fixation as a more controlled release of N (since legumes release N slowly through mineralization rather than the immediate availability of a fertilizer application). Climate policies aiming to cut N₂O emissions (as part of national greenhouse gas inventories) further justify the shift. In sum, various environmental regulations create a context where farmers are looking for ways to maintain yields with less fertilizer – legumes with rhizobia offer one proven solution.
Overall, EU sustainability goals and the supporting policy instruments strongly favor the increased use of Rhizobium-legume systems. We see a convergence of economic motives (protein independence, input cost reduction) and environmental motives (emission cuts, biodiversity gains) leading to a renaissance of interest in legumes. The inclusion of Rhizobium inoculants in the official “toolbox” (via the EU Fertilising Products Regulation) means it’s easier to commercialize and trade these biofertilizers across the EU, facilitating adoption. As these policies are implemented, it’s likely that the area under legumes will expand and the practice of inoculation will become more routine even in regions where it hasn’t been common for decades. The net effect anticipated is a more climate-smart, sustainable agriculture where a significant share of nitrogen needs is met biologically.
Conclusion and Outlook
Rhizobium species, through their symbiotic relationship with legumes, provide a natural, sustainable source of nitrogen that is foundational for climate-resilient agriculture. Biologically fixed nitrogen can replace a substantial portion of synthetic fertilizers, thereby lowering costs for farmers and mitigating environmental impacts such as greenhouse gas emissions and water pollution. Beyond nitrogen, Rhizobium symbiosis enhances crop yields, quality (especially protein content), and even plant health by fortifying stress and disease tolerance. In the soil, the legacy of Rhizobium and legumes is improved fertility and a richer microbiome, which benefit subsequent crops and overall farm ecosystem function.
Adopting Rhizobium-based solutions is not without its challenges – appropriate matching of strains to crops, attention to soil conditions, and ensuring high-quality inoculants are all crucial. However, decades of research and farmer experience have equipped us with the knowledge to navigate these challenges. In practice, successful case studies from around the world (including Europe) show that with the right management, inoculated legumes can thrive in diverse agroecological zones, from smallholder fields to large-scale commercial farms.
The future outlook for Rhizobium in agriculture is very promising. On the research front, there are continuing efforts to improve the efficiency of biological nitrogen fixation. This includes breeding legume varieties that can fix more nitrogen or nodulate more under suboptimal conditions, and bioengineering rhizobia that are more effective or that can extend their host range. One cutting-edge area is attempting to transfer the Rhizobium-legume symbiosis to non-legume crops (like engineering cereal crops to form nodules) – a challenging goal, but if ever realized, it could revolutionize crop production. In the nearer term, selecting elite rhizobial strains for each environment (e.g., drought-proof rhizobia for arid lands, cold-tolerant ones for high latitudes) will help maintain fixation in the face of climate change.
From a farming systems perspective, we may see more integrated approaches: multi-species cover cropping where legumes are included to fix N, or intercropping systems (such as cereals grown in mixture with a legume) to share nitrogen via root zone interactions. Rhizobium will be a key player in such regenerative practices. The development of consortia products (rhizobia combined with other beneficial microbes) is another trend that could amplify benefits – essentially packing more functionality (nitrogen fixation, phosphate solubilization, disease suppression) into one inoculum package.
Policy drivers, especially in Europe, are aligning to support these biological solutions. If the EU achieves its target of 25% of farmland under organic farming by 2030 and a significant reduction in synthetic fertilizers , it will be largely thanks to natural processes like Rhizobium BNF filling the gap.
We can expect increased extension efforts and knowledge transfer to ensure farmers know how to utilize Rhizobium optimally. This includes training on inoculation techniques, soil health management, and showcasing demonstrative successes (which builds farmer confidence in these methods).
In conclusion, Rhizobium species represent a cornerstone of sustainable agriculture – a powerful example of a biological solution to an agronomic problem. Their ability to secure nitrogen from the air and feed it to plants is nothing short of ecological engineering, honed by evolution and now harnessed by modern farming. Embracing and enhancing this symbiosis allows us to move away from over-reliance on chemical fertilizers, thereby making agriculture more environmentally friendly and resilient to climate and economic fluctuations.
Sources: The information in this report is supported by extensive scientific literature and field studies on Rhizobium-legume symbiosis and its agricultural impacts, including:
Nodulation mechanism details (16.5G: The Legume-Root Nodule Symbiosis - Biology LibreTexts), symbiosis benefits for crop yield/quality (Frontiers | Rhizobium Impacts on Seed Productivity, Quality, and Protection of Pisum sativum upon Disease Stress Caused by Didymella pinodes: Phenotypic, Proteomic, and Metabolomic Traits) (Frontiers | Grain Legume Yield Responses to Rhizobia Inoculants and Phosphorus Supplementation Under Ghana Soils: A Meta-Synthesis), soil health and disease resistance observations (Frontiers | Rhizobium Impacts on Seed Productivity, Quality, and Protection of Pisum sativum upon Disease Stress Caused by Didymella pinodes: Phenotypic, Proteomic, and Metabolomic Traits), greenhouse gas mitigation potential (Enhancing Rhizobium–Legume Symbiosis and Reducing Nitrogen Fertilizer Use Are Potential Options for Mitigating Climate Change) (New Insights into the Use of Rhizobia to Mitigate Soil N 2 O Emissions), and European policy frameworks promoting legumes and biological nitrogen fixation (Valorisation of ecosystem services provided by legume crops | Horizon-europe.gouv.fr) (Nature and Nitrogen - CAP battles over Conditionality), among other referenced studies. These illustrate the multifaceted value of Rhizobium in advancing sustainable agriculture.
Comments